
Research Fields
At the Biomolecular Imaging and NanoPhotonics Lab we develop optical methods and novel bio sensors for fluorescence-based biomolecular and biomedical imaging.
Fluorescent molecules emitting at wavelengths in the physiologically relevant “water window” (700−900 nm), as well as in the shortwave infrared (SWIR) regime, are of particular interest due to the large penetration depth of near-infrared (NIR) light in most biological media and offer the potential for imaging at significant depths in living tissues. Yet, Achieving bright fluorescent emission with photostable and biocompatible near-IR fluorophores has proven to be extremely difficult. In our team, we aim to develop optical-based methods and optical nanosensors for biomolecular targets and biomedical theranostics using nanoparticles, to probe and imaging living systems at the nano-scale. The methods that will be developed will enable fast and sensitive imaging of cell tissues, using unique super-fast cameras for real-time and vide rate imaging.
​
Fluorescence lifetime imaging (FLIM)
We aim to develop widefield FLIM in the NIR regime for biomedical applications in vivo. We are using a large (512x512 pixels) time-gated SPAD array which enables us to calculate the fluorescence lifetime of each pixel in the image, in real-time, with a very high sensitivity. Thus, for example, it will be possible to quickly identify molecular species mixtures, monitor protein-protein interactions (FRET), cells tracking in immunotherapy treatments, and more. This imaging ability is crucial both for understanding basic biological processes and for drug discovery and development.
​
​
​
​
​
Encapsulated novel Cyanine dyes
Cyanine dyes are important fluorescent building blocks commonly used for protein labeling, and cell imaging. The advantages of cyanine dyes include that their absorption and emission maxima are found in the near infrared and shortwave infrared light (SWIR) (800–1500 nm). In this region, absorption, auto fluorescence, and scattering in biological tissue are minimal. This is especially important when in vivo experiments are performed alongside the imaging of biological tissues in preclinical investigations. We aim to develop a novel encapsulated highly SWIR cyanines fluorescent dyes. In dyes such as cyanines, neighboring chromophores are oriented in a head-to-tail fashion, leading to J-aggregate which can shift their absorption band to the desired shortwave infrared (SWIR) part of the spectrum, in which biological samples are almost transparent to irradiated light.
​
​
​
​
​
Metal enhanced fluorescence
It has long been known that, in the proximity of a metallic surface, fluorescence emission of molecules can be enhanced; this is also the case for metallic nanostructures and nanoparticles adjacent to a fluorophore. The presence of a nearby metallic nanoparticle can not only enhance the quantum yield but also stabilize adjacent fluorophores against photobleaching. Understanding precisely how metallic nanostructures enhance molecular fluorescence is of general fundamental interest and may ultimately provide practical routes to enhancing light emission from a variety of materials systems and devices far beyond the specific application of bioimaging. Metallic nanoparticles have been shown to enhance the fluorescence emission and decrease the molecular excited-state lifetimes of vicinal fluorophores. The fluorescence enhancement is attributable to a combination of processes including enhanced absorption by the molecule, modification of the radiative decay rate of the molecule, and enhanced coupling efficiency of the fluorescent emission to the far field.
​
​
Modeling light-tissue Interaction
The basis for the development of optical-based diagnosis methods lies in the theory of light transfer in biological tissues. The optical regime is highly desired for biomedical applications as it is a nonionizing radiation, abundantly available, and inexpensive. Several approaches have been developed in order to best describe the photon transfer in biological tissues, when light scattering in living tissue is in the basis of these approaches. However, scattering in near-infrared region is weaker than that in the visible region therefore, it is of highly importance to investigate the behavior of excitation and emission photons in near-infrared fluorescence within tissues, which could be applied for in vivo imaging. We aim to study and rely on multi-layered fluorescence Monte Carlo model of in vivo fluorescence based imaging, for both visible and near-infrared light. Fluorophore concentration and quantum yield are important parameters which influence the mechanism of fluorescence onset, when the depths and sizes of a fluorescent target embedded in the tissue model are of interest.


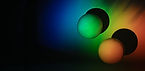